|
 |
June 2025
GAMMA-400 scientific complex
|
|
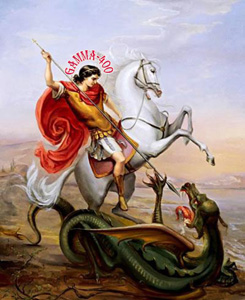 |
|
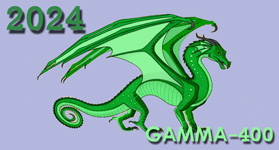 2024
|
|
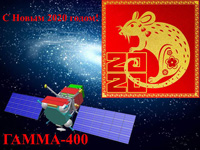 2020 |
|
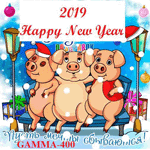 2019 |
|
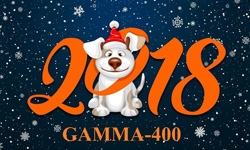 2018 |
| 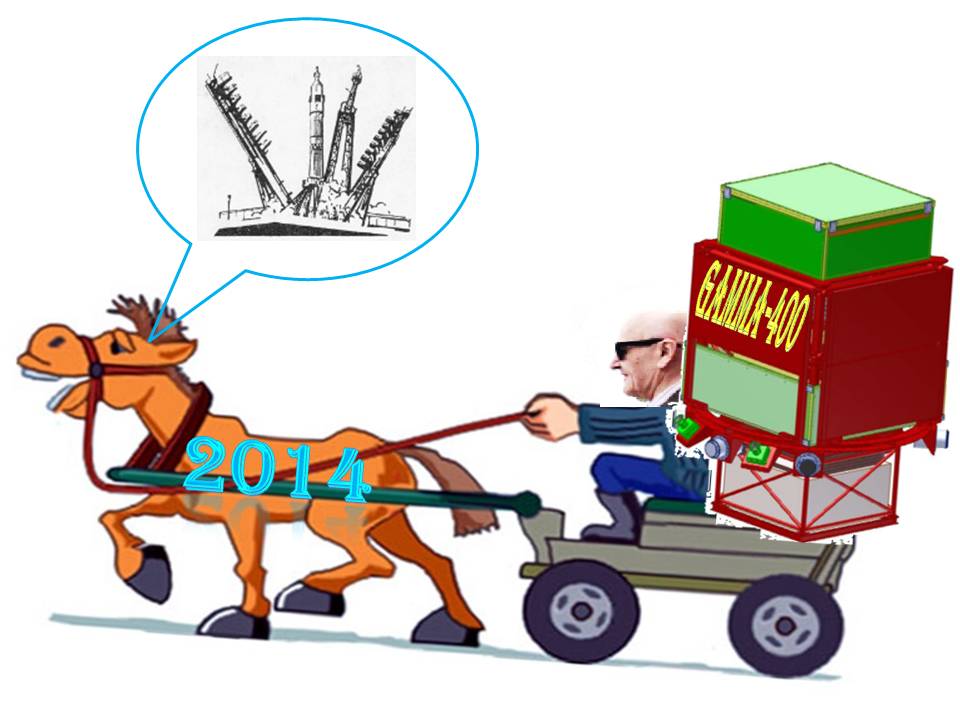 2014 |
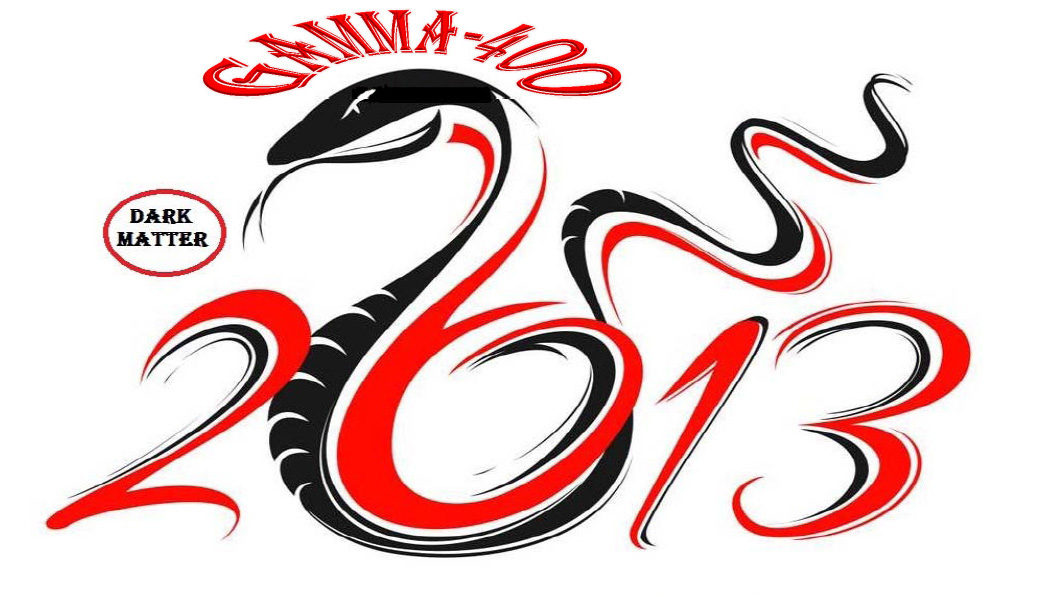 2013 |
|
Roscosmos TV studio: GAMMA-400,
Space environment" no. 290 on July 15, 2020.
More details on the site: www.tvroscosmos.ru.
GAMMA-400 (Gamma Astronomical Multifunctional Modular Apparatus) scientific complex is designed to obtain the data for determining the dark matter nature in the universe, to develop the theory of origin of high-energy cosmic rays and the elementary particle physics, to investigate cosmic gamma-ray emission in the high-energy range of 20 MeV – 1000 GeV and X-ray emission in the energy range of 5-30 keV, to detect cosmic rays, and to search for and study gamma-ray bursts.
The GAMMA-400 design and investigations are performed within the framework of the Russian Federal Space Programs for 2006-2015 and 2016-2025.
The GAMMA-400 scientific complex is designed by:
GAMMA-400 Principal Investigator is Professor Arkadiy Galper;
GAMMA-400 Deputy Principal Investigator, Project Manager and Chief Designer is Nikolay Topchiev.
The GAMMA-400 Project was approved in 2009 by academicians Vitaly Ginzburg and Gennady Mesyats.
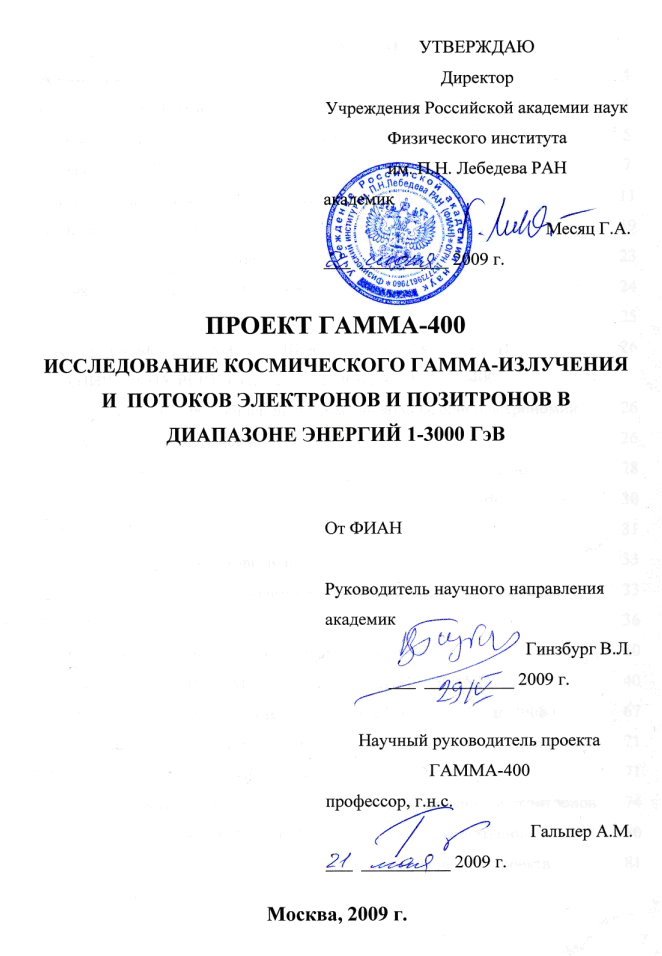
GAMMA-400 scientific complex
The GAMMA-400 gamma-ray telescope and the ART-XC X-ray telescope located coaxially, as well as magnetic plasma detectors located on remote booms are installed on the Navigator space platform. Long-term simultaneous observations of astrophysical objects are carried out.
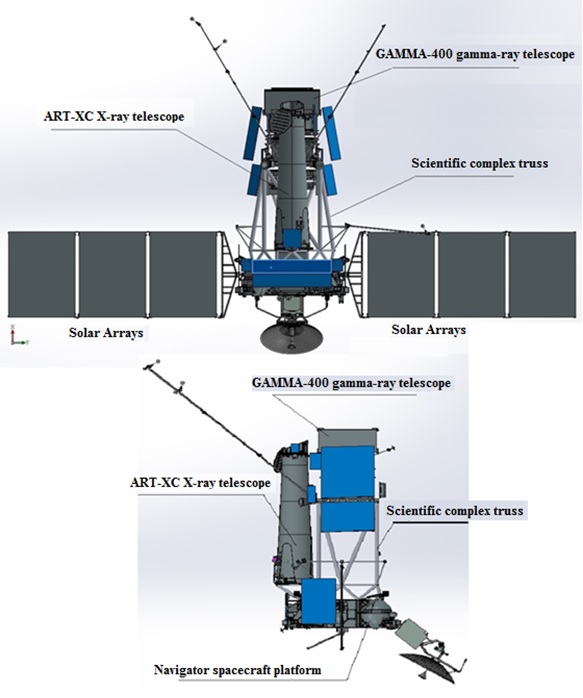
Scientific goals of the GAMMA-400 gamma-ray telescope
The current state of fundamental studies in cosmology, astronomy, physics of high-energy particles and cosmic rays poses a number of problems that cannot be solved without attracting the results of research on extra-atmospheric gamma-ray astronomy of high energies (108-1012 eV), as well as the simultaneous study of high-energy electron-positron component of galactic cosmic rays. On the basis of current results of gamma-ray astronomy, the results of studies of the nature of dark matter, as well as researches on the GAMMA-400 project, the main scientific goals of the GAMMA-400 gamma-ray telescope are determined:
1. Measurement of energy spectra of galactic and extragalactic diffuse and isotropic gamma-ray emission. Search for anomalies in gamma-ray spectra. Search for gamma-ray lines in the emission of discrete gamma-ray sources, in diffuse and isotropic gamma-ray emission, arising from the annihilation and decay of the components of dark matter.
2. Detection of fluxes of electrons + positrons, measurement of the energy spectra of these particles, searching for the features in their spectra that could be associated with the processes of annihilation and decay of the components of dark matter.
3. Search for new and study of known galactic and extragalactic discrete sources of high-energy gamma-ray emisssion: supernova remnants, pulsars, accreting objects, microquasars, galaxies with active nuclei, blazars, quasars; measurement of their energy spectra and luminosity.
4. Identification of discrete gamma-ray sources with known sources of the emission in other energy ranges, including discrete sources recorded by ground-based gamma-ray telescopes.
5. Monitoring the luminosity and energy spectrum of high-energy gamma-ray sources to study the nature of their variability.
6. Searching for and studying gamma-ray bursts.
7. Detection of high-energy gamma-ray emission and fluxes of electrons + positrons from solar flares.
GAMMA-400 gamma-ray telescope
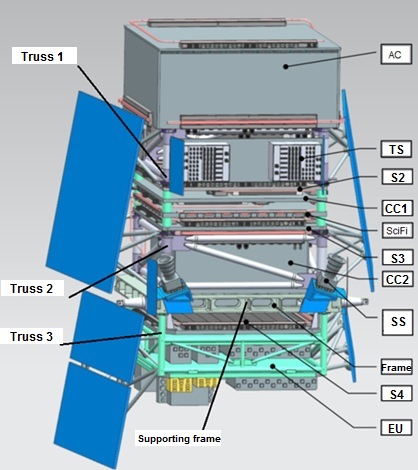
Physical scheme of the GAMMA-400 gamma-ray telescope
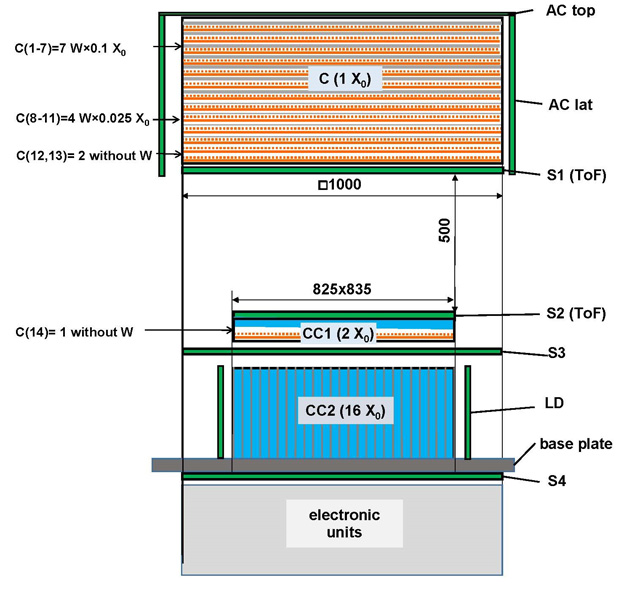
GAMMA-400 gamma-ray telescope structure
GAMMA-400 gamma-ray telescope consists of:
- top (AC top) and lateral (AC lat) anticoincidence detectors;
- converter-tracker (C) from 13 planes of two-layer (with mutually perpendicular arrangement) SciFi coordinate detectors: 7 planes interlayered with tungsten W with a thickness of 0.1 X0; 4 planes interlayered with W a thickness of 0.025 X0; 2 planes without tungsten;
- time-of-flight system (TOF) of scintillation detectors S1 and S2 spaced 500 mm apart;
- coordinate-sensitive calorimeter (CC) from CC1, CC2, LD CC2:
a) CC1 consists of a layer of CsI (Tl) crystals and 1 plane of two-layer (with mutually perpendicular arrangement) SciFi coordinate detectors without W;
b) CC2 consists of CsI (Tl) crystals;
c) lateral detectors of CC2 (LD CC2);
- scintillation detectors S3 and S4.
Additionally, the gamma-ray telescope includes:
- trigger system (TS), scientific data collection system (SDCS) and other electronic units (EUs);
- 2 star sensors.
Particle detection
Primary gamma rays pass through the anticoincidence detectors AC without interaction. Charged particles are excluded from detection by anticoincidence detectors. Gamma rays are converted into an electron-positron pair in the converter-tracker C. Electron-positron pair, when interacting with the matter of the detectors, creates an electromagnetic shower, which is recorded in the detectors of the gamma-ray telescope. The time-of-flight system TOF forms the aperture of the gamma-ray telescope and excludes particles from the lower hemisphere from detection. An electromagnetic shower is recorded in two parts of the CC1 and CC2 calorimeter. Shower particles are recorded in S3 and S4 scintillation detectors.
Particle detection is carried out by two apertures: from top to bottom is the main one formed by the AC and ToF, and the lateral one formed by the LD CC2 detectors and the CC2 calorimeter.
High-energy (more than several GeV) particles interacting with the calorimeter matter create a flux of particles (mainly photons with energies up to 1 MeV), which move from the calorimeter to the AC (the so-called "backsplash particles") and can interact in AC and exclude from recording primary particles. To exclude the detection of these particles, both segmentation (all scintillation detectors are two-layer and consist of separate strips) and temporal (time-of-flight) methods are used.
Improvement of the angular resolution is achieved by high-precision determination of the conversion point in the multilayer converter-tracker and reconstruction of the shower axis using SciFi coordinate detectors in the converter-tracker C and the CC1 calorimeter. This method allows us to achieve the highest angular resolution of ~0.01° at energies of ~100 GeV.
The use of a thick calorimeter (up to ~18 X0) makes it possible to expand the energy range of recorded particles to several TeV for gamma rays and up to ~10 TeV for electrons + positrons and increase the energy resolution of the gamma-ray telescope to ~2% at energies of ~100 GeV.
Proton rejection of ~5×105 is achieved by rejection both in the calorimeter and in other detectors.
Table 1. Key performance of the GAMMA-400 gamma-ray telescope
|
Phase A
(first stage)
2009-2010
|
Phase A
(second stage)
2011-2012
|
Phase A
(third stage)
2013-2014
|
Phase A
(forth stage)
2016-2021
|
Angular resolution
(Eγ > 100 GeV)
|
0.2°
|
~0.01°
|
~0.01°
| ~0.01° |
Energy resolution
(Eγ > 10 GeV)
|
~3%
|
~1%
|
~1%
| ~2% |
Energy range - gamma rays
- electrons+positrons
|
30-1000 GeV
|
0.1-3000 GeV
|
0.1-3000 GeV
| 0,02-1000 GeV
0,02-10000 GeV
|
Sensitive area
|
0.44 m2
|
0.64 m2
|
1.0 m2
| ~0,7 m2 |
Telemetry downlink volume
|
500 Mbytes/day
|
100 Gbytes/day
|
100 Gbytes/day
|
100 Gbytes/day |
Particles
|
gamma rays,
electrons+positrons, protons, nuclei
|
gamma rays,
electrons+positrons, protons, nuclei
|
gamma rays,
electrons+positrons, protons, nuclei
|
gamma rays, electrons+positrons |
Table 2. Comparison of the Fermi-LAT and GAMMA-400 performance
|
Fermi-LAT
|
GAMMA-400
|
Orbit
|
560 km
|
500-300000 km
|
Energy range
|
100 MeV - 300 GeV
|
20 MeV - 1000 GeV
|
Sensitive area
|
1.8 m2
|
0.7 m2
|
Coordinate detectors
|
Si strips
with pitch 0.23 mm
|
SciFi
with pitch 0.2 mm
|
Angular resolution
(Eγ > 100 GeV)
|
~0.1°
|
~0.01°
|
Calorimeter
- thickness, X0
|
CsI(Tl)
8.5
|
CsI(Tl) + SciFi
~18
|
Energy resolution
(Eγ > 10 GeV)
|
~10%
|
~2%
|
Proton rejection factor
|
104
|
~5×105
|
Telemetry downlink volume, Gbytes/day
|
20
|
100
|
Table 3. Comparison of performance for current space-based gamma-ray telescopes and spectrometers with GAMMA-400
|
AGILE
|
Fermi-LAT
|
CALET
|
DAMPE
|
GAMMA-400
|
Operation period
|
2007–present
|
2008–present
|
2015–present
|
2015–present
|
~2030
|
Energy range,
GeV
|
0.03-50
|
0.1-300
|
10-10000
|
1–10000
|
0.02–1000
|
Operation mode
|
Scanning
|
Scanning
|
Scanning
|
Scanning
|
Point-source
|
Effective area, m2
|
0.05
(1 GeV)
|
0.9
|
0.06
|
0.12
(100 GeV)
|
~0.4
(100 GeV)
|
Angular resolution
|
0.2º
(10 GeV)
|
0.1º (100 GeV)
|
0.2º (100 GeV)
|
0.1º (100 GeV)
|
0.01º (100 GeV)
|
Energy resolution, %
|
100
(0,4 GeV)
|
~10
(100 GeV)
|
~2
(100 GeV)
|
~2
(100 GeV)
|
~2
(100 GeV)
|
On-axis calorimeter thickness, X0
|
1.5
|
8.5
|
30
|
32
|
18
|
Table 4. Comparison of performance for future space-based gamma-ray telescopes and spectrometers with GAMMA-400
|
e-ASTROGAM
|
AMEGO
|
HERD
|
AMS-100
|
GAMMA-400
|
Operation period
|
~2028
|
~2029
|
~2027
|
~2039
|
~2030
|
Energy range, GeV
|
3·10-4 – 3
|
2·10-4 – 10
|
0.5 – 105
|
0.1 – 104
|
0.02 - 1000
|
Operation mode
|
Scanning
|
Scanning
|
Scanning
|
Scanning
|
Point-source
|
Effective area, m2
|
0,16
(1 GeV)
|
0,3
(1 GeV)
|
~0,8
|
~2,5
|
~0,4
(100 GeV)
|
Angular resolution
|
0,2°
(1 GeV)
|
2°
(100 GeV)
|
0,1°
(10 GeV)
|
~0,01°
(100 GeV)
|
0,01°
(100 GeV)
|
Energy resolution, %
|
30
(100 MeV)
|
10
(1 GeV)
|
1-2
(200 GeV)
|
1-2
(100 GeV)
|
~2
(100 GeV)
|
On-axis calorimeter thickness, X0
|
4.3
|
5
|
55
|
70
|
18
|
GAMMA-400 spacecraft
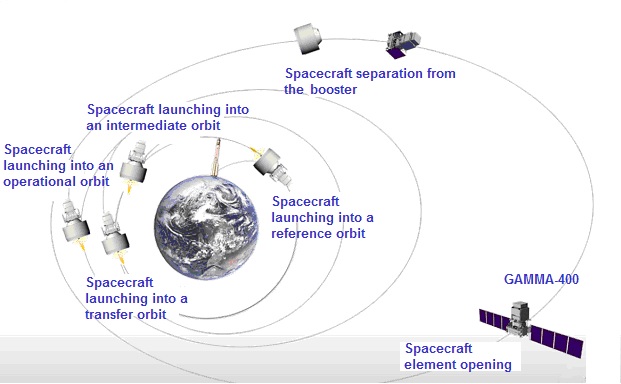
The GAMMA-400 spacecraft launching scheme.
The GAMMA-400 spacecraft and Navigator service module are designed by Lavochkin Association.
|